alberchico
I really should change my personal text
- Joined
- 14 January 2014
- Messages
- 708
- Reaction score
- 1,514
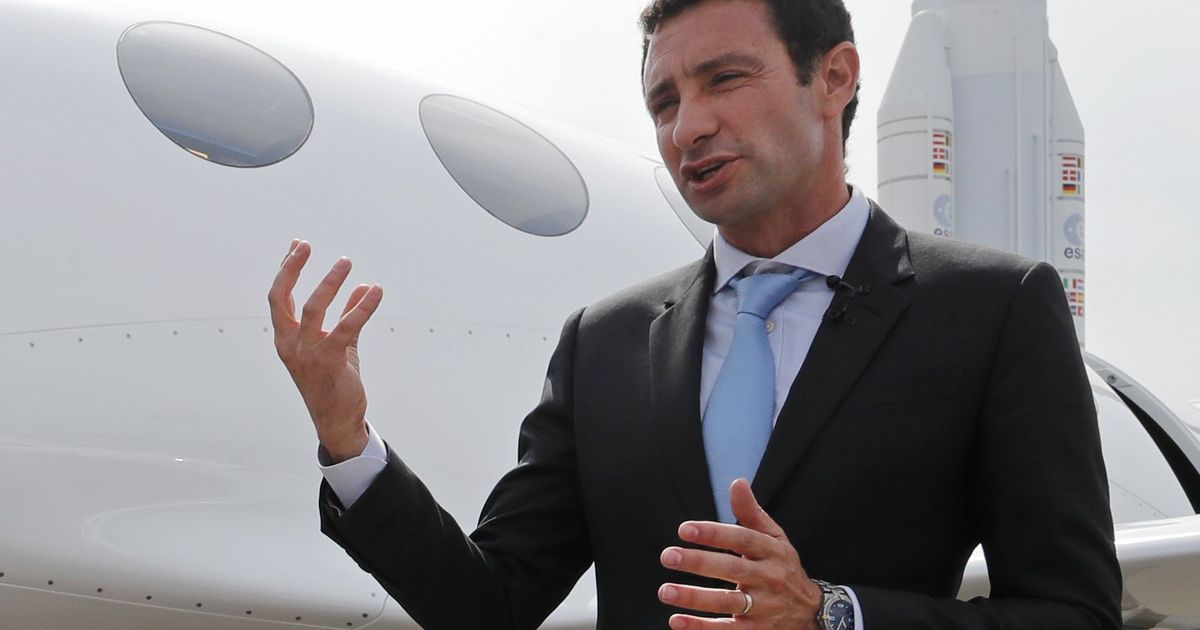
Electric airplane CEO ousted ahead of 1st flight
Omer Bar-Yohay, co-founder and CEO of electric aircraft company Eviation, abruptly stepped down Monday, weeks ahead of a planned first flight.

The timing is so odd. You would think he would have stayed on board for just a few more days to oversee the first flight and handle all the media appearances that would be required of the company. He had been with Eviation since the very beginning and had been doing an effective job promoting the company.
Edit: based on this article it appears that he was ousted by the board. Mabye they realized that they had been misled regarding the exact capabilities of the aircraft regarding range as well as future battery improvements. The article I mentioned in my last post claims that this project has serious range deficiencies and that Eviation is basically lying while quietly hoping that improving battery tech will catch up and resolve those issues.
I would also like to point out that on Jan 6 another top executive, who had senior leadership roles at both Eviation and Magnix, which are owned by the same conglomerate, also abruptly stepped down. Something is going on.
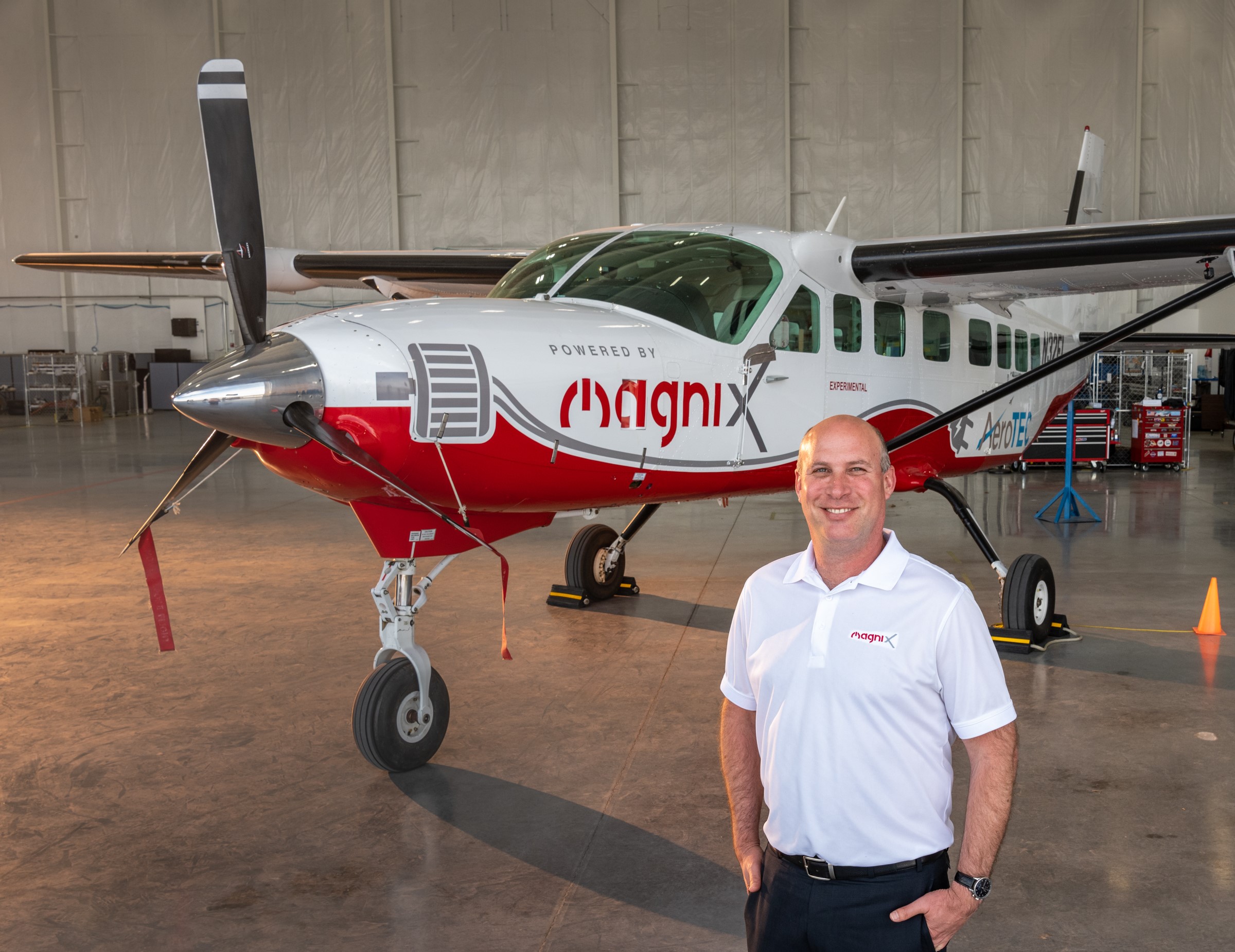
Electric aviation executive Roei Ganzarski steps down from leadership roles at MagniX and Eviation
Roei Ganzarski, the former Boeing manager who went on to give a boost to all-electric aviation, is leaving his executive posts at MagniX and Eviation Aircraft.

Last edited: